The newly discovered brain signal which may be a key to being human
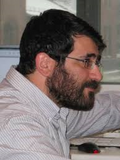
Scientists around Albert Gidon (pic.) and Matthew Larkum of the Institute for Biology of the Humboldt University in Berlin announce in Science the discovery of a yet unknown form of brain cell messaging which seems unique to humans, and which greatly increases the information processing power of the brain. The Greek Foundation for Research and Technology - Hellas (IMBB-FORTH) in Crete also contributed to the research.
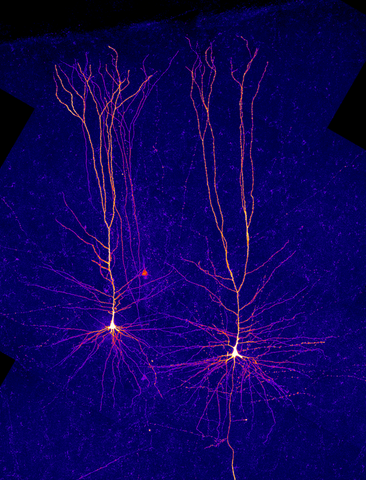
These are neurons of the 2/3 layer of the human neo-cortex
Cells nestled in the outermost layers of the human brain generate a special kind of electrical signal that might grant them an extra boost of computing power, new research suggests. What's more, this signal may be unique to humans — and may explain our unique intelligence, according to the study authors.
Brain cells, or neurons, link up through long, branching wires and shuttle messages along these cables to communicate with each other. Each neuron has both an outgoing wire, called an axon, and a wire that receives incoming messages, known as a dendrite. The dendrite passes on information to the rest of the neuron through bursts of electrical activity. Depending on how the brain is wired up, each dendrite may receive hundreds of thousands of signals from other neurons along its length. While scientists believe these electrical spikes help wire the brain and may underlie abilities like learning and memory, the exact role of dendrites in human cognition remains a mystery.
Now, researchers have uncovered a new flavor of electrical spike in human dendrites — one they think might allow the cells to perform computations once thought too complex for a single neuron to tackle on its own. The study, published Jan. 3 in the journal Science, notes that the newfound electrical property has never been observed in any animal tissue other than human, raising the question of whether the signal uniquely contributes to human intelligence, or to that of primates, our evolutionary cousins.
Matthew Larkum plays Tchaikovsky
Up until now, most dendrite studies have been carried out in rodent tissue, which shares basic properties with human brain cells, said study co-author Matthew Larkum, a professor in the department of biology at Humboldt University in Berlin. However, human neurons measure about twice as long as those found in a mouse, he said.
"That means the electrical signals have to travel twice as far," Larkum told Live Science. "If there was no change in the electrical properties [between rodents and people], then that would mean that, in the humans, the same synaptic inputs would be quite a bit less powerful." In other words, electrical spikes received by a dendrite would weaken significantly by the time they reached the cell body of the neuron.
So Larkum and his colleagues set out to uncover the electrical properties of human neurons to see how these longer dendrites actually manage to send signals effectively.
First, the researchers had to get their hands on human brain tissue samples, a notoriously scarce resource. The team ended up using neurons that had been sliced from the brains of epilepsy and tumor patients as part of their medical treatment. The team focused on neurons resected from the cerebral cortex, the wrinkled exterior of the brain that contains several distinct layers. In humans, these layers hold dense networks of dendrites and grow to be extremely thick, an attribute that may be "fundamental to what makes us human," according to a statement from Science.
"You get the tissue very infrequently, so you've just got to work with what's in front of you," Larkum said. And you have to work fast, he added. Outside the human body, the oxygen-starved brain cells only remain viable for about two days. To take full advantage of this limited time window, Larkum and his team would gather measurements from a given sample for as long as they could, sometimes working for 24 hours straight.
During these experimental marathons, the team chopped brain tissue into slices and poked holes in the dendrites contained within. By sticking thin glass pipettes through these holes, the researchers could inject ions, or charged particles, into the dendrites and observe how they changed in electrical activity. As expected, the stimulated dendrites generated spikes of electrical activity, but these signals looked very different from any seen before.
Each spike ignited for only a brief period of time — about a millisecond. In rodent tissue, this type of supershort spike occurs when a flood of sodium enters a dendrite, triggered by a particular accumulation of electrical activity. Calcium can also trigger spikes in rodent dendrites, but these signals tend to last 50 to 100 times longer than sodium spikes, Larkum said. What the team saw in human tissue, though, seemed to be a strange hybrid of the two.
"Although it looked like a sodium event, it was actually a calcium event," Larkum said. The team members tested what would happen if they prevented sodium from entering their sample dendrites and found that the spikes continued to fire unabated. What's more, the supershort spikes fired in rapid succession, one right after the other. But when the researchers blocked calcium from entering the neurons, the spikes stopped short. The scientists concluded that they had stumbled upon a brand-new class of spike, one similar in duration to sodium but controlled by calcium.
"These [spikes] look different than whatever we have known so far from other mammals," said Mayank Mehta, a professor in the departments of neurology, neurobiology physics and astronomy at the University of California, Los Angeles, who was not involved in the study. The big question is, how do these spikes relate to actual brain function, he said. (...)
Nicoletta Lanese
Read the whole article in LiveScience
Dendrites are the traffic lights of our nervous systems
Brains – especially those of the human variety – are often compared to computers. The analogy has its limits, but on some levels they perform tasks in similar ways.
Both use the power of an electrical voltage to carry out various operations. In computers it's in the form of a rather simple flow of electrons through intersections called transistors.
In neurons, the signal is in the form of a wave of opening and closing channels that exchange charged particles such as sodium, chloride, and potassium. This pulse of flowing ions is called an action potential.
Instead of transistors, neurons manage these messages chemically at the end of branches called dendrites.
"The dendrites are central to understanding the brain because they are at the core of what determines the computational power of single neurons," Humboldt University neuroscientist Matthew Larkum told Walter Beckwith at the American Association for the Advancement of Science.
Dendrites are the traffic lights of our nervous system. If an action potential is significant enough, it can be passed on to other nerves, which can block or pass on the message.
This is the logical underpinnings of our brain – ripples of voltage that can be communicated collectively in two forms: either an AND message (if x and y are triggered, the message is passed on); or an OR message (if x or y is triggered, the message is passed on).
Arguably, nowhere is this more complex than in the dense, wrinkled outer section of the human central nervous system; the cerebral cortex. The deeper second and third layers are especially thick, packed with branches that carry out high order functions we associate with sensation, thought, and motor control. (...)
Mike McCrae
Read the whole article in Science Alert